According to some critics, nuclear fusion as an energy source is always thirty years in the future. Fast computer models that predict heat dissipation are one of the keys to making this futuristic music sound like it could one day.
Where is the hottest place in our solar system? Many people think it’s the Sun, but that’s not true. Almost every day, nuclear fusion experiments around the world reach temperatures of around 100 million degrees Celsius, roughly ten times warmer than the core of the Sun. Scientists are using these experiments to better understand nuclear fusion as an intermediate step toward building reactors that can generate electricity. The current experiments are being conducted in reactor vessels that are shaped similarly to future power-producing reactors, but are more than twice as small. The opening image shows the UK’s JET company, which holds the world record for fusion power. The JET reactor vessel is shaped like a doughnut, about eight meters in diameter.
Although current experiments simulate the conditions of future reactors as closely as possible, the fusion power produced is still limited. This keeps the thermal load on the reactor wall low enough that the wall does not melt. If we are to use fusion to generate electricity, the fusion power produced must be greatly increased. Some research inThermal and Fluid EngineeringThe KU Leuven group is part of the enormous challenge of ensuring that the reactor vessel does not melt at these high forces.
How does nuclear fusion work?
Nuclear fusion is the source of the Sun’s energy. To recreate the Sun on Earth, we use two isotopes of hydrogen: deuterium and tritium. Both atomic nuclei contain one positively charged proton. Deuterium also has one neutron, while tritium has two neutrons. When the nuclei fuse, helium is formed and a neutron is released.
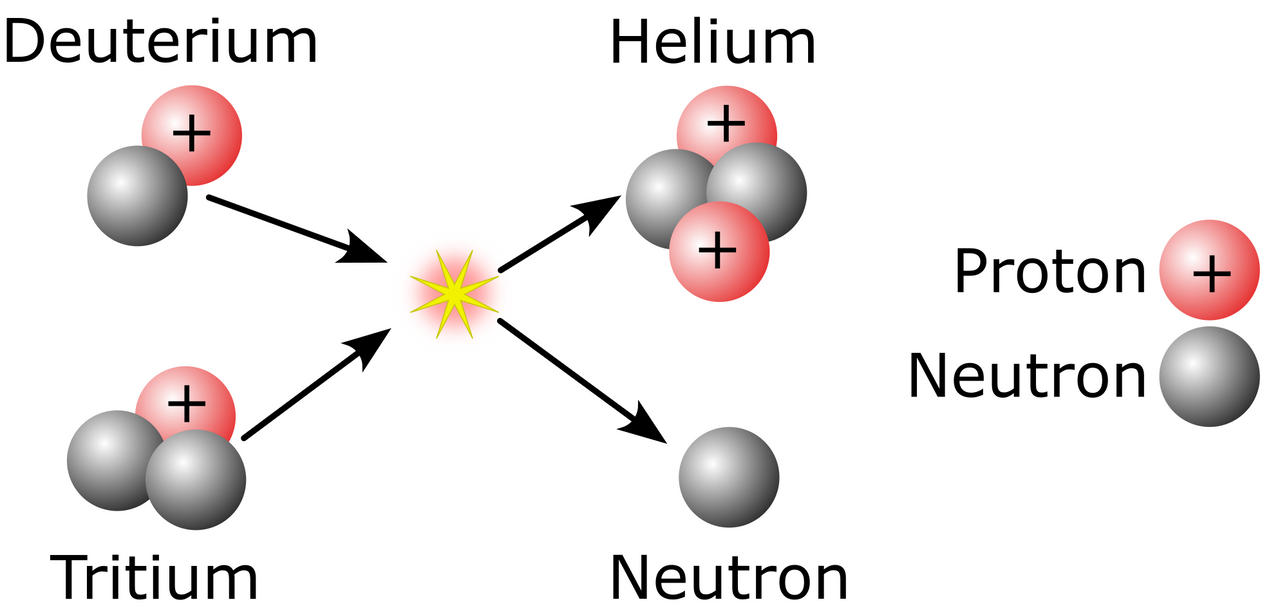
During this fusion process, part of the mass (M) is lost. This is achieved through Einstein’s famous formula, H = Mouloudia2, Convert it into energy (H). Because the speed of light (G = 300,000 km/s) is a tremendous speed, any small decrease in mass results in a tremendous amount of energy. Half a bath of seawater and the tritium that can be made from the lithium in a laptop battery is enough to power one person for thirty years. Unlike nuclear fission, which is the process that takes place in current nuclear reactors, the neutrons released during nuclear fusion do not lead to further fusion reactions. This makes nuclear fusion safe. Furthermore, nuclear fusion produces only a limited amount of short-lived radioactive waste, and no long-lived waste.
very high temperatures
Because the positively charged nuclei used in a nuclear fusion reaction repel each other strongly, the temperature in the reactor must be very high. At this high temperature, the particles have enough energy to get close enough together. You can roughly compare this to throwing a ball over a high net. If you don’t give the ball enough speed, it won’t clear the net. In the case of the repulsion between two positively charged nuclei, this effect is even more extreme than in the ball, because the force of repulsion increases dramatically as the nuclei get closer together. This is similar to bringing the positive poles of two magnets closer together.
“At extremely high temperatures, plasma is created, which is a mixture of ions and electrons.”
Although atomic nuclei are positively charged, atoms are neutral because of the presence of negatively charged electrons (blue molecules in the figure below). At temperatures well below 10,000 degrees Celsius, deuterium and tritium atoms form molecules, and you get the figure on the left. If you increase the temperature, the molecular bonds first break (middle figure), and eventually the electrons become freed from the atoms (right figure). This combination of positive ions and negative electrons becomes plasma His name. The temperature of nuclear fusion is 150 million degrees Celsius. plasma It was created.
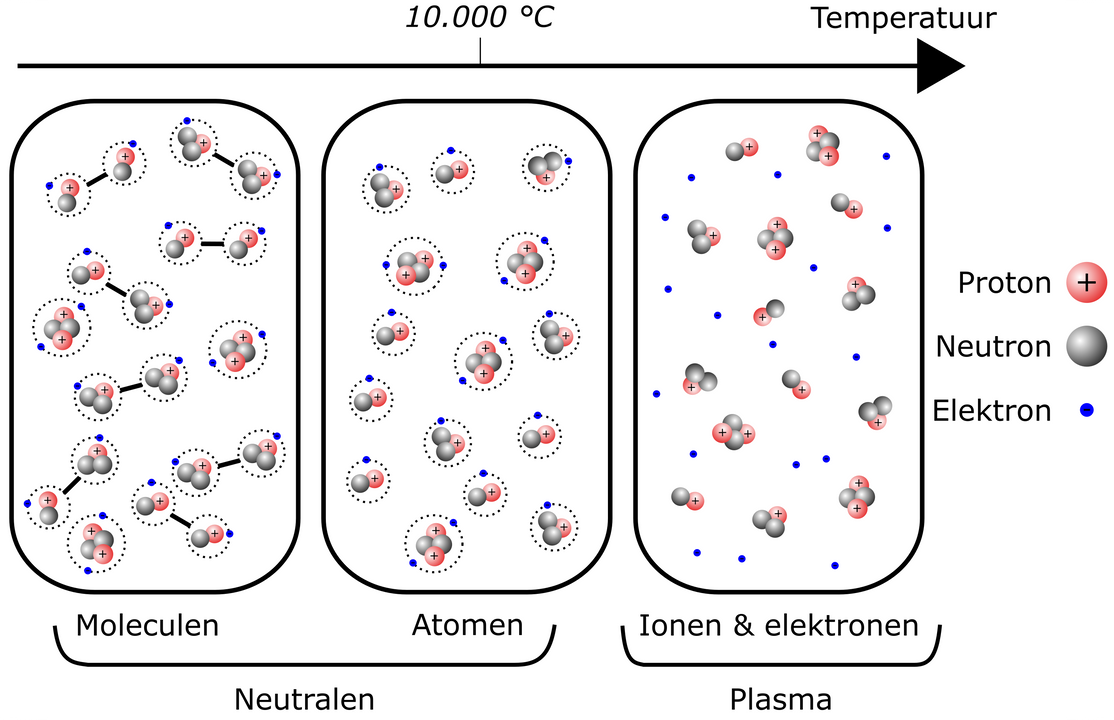
Heat dissipation problem
We can trap charged particles using magnetic fields, so that they become very hot. plasma It does not come into direct contact with the reactor wall. This usually occurs in a donut-shaped reactor, known as a Tokamakas shown in the opening image.
After a certain period of time, the plasma particles are no longer magnetically confined and fly at great speed to a small part of the wall (see figure below). Because the surface area on which the particles land is so small, the thermal load is enormous. This thermal load is expressed in megawatts (MW) per square meter (m2).2). if it was plasma If it flows unimpeded to this small contact surface, we can easily get a heat load of more than 50 MW/m.2 (Left section position.) For comparison, a typical spacecraft thermal load upon re-entry into the atmosphere is 1 MW/m², which gives an idea of the catastrophic consequences for the reactor.
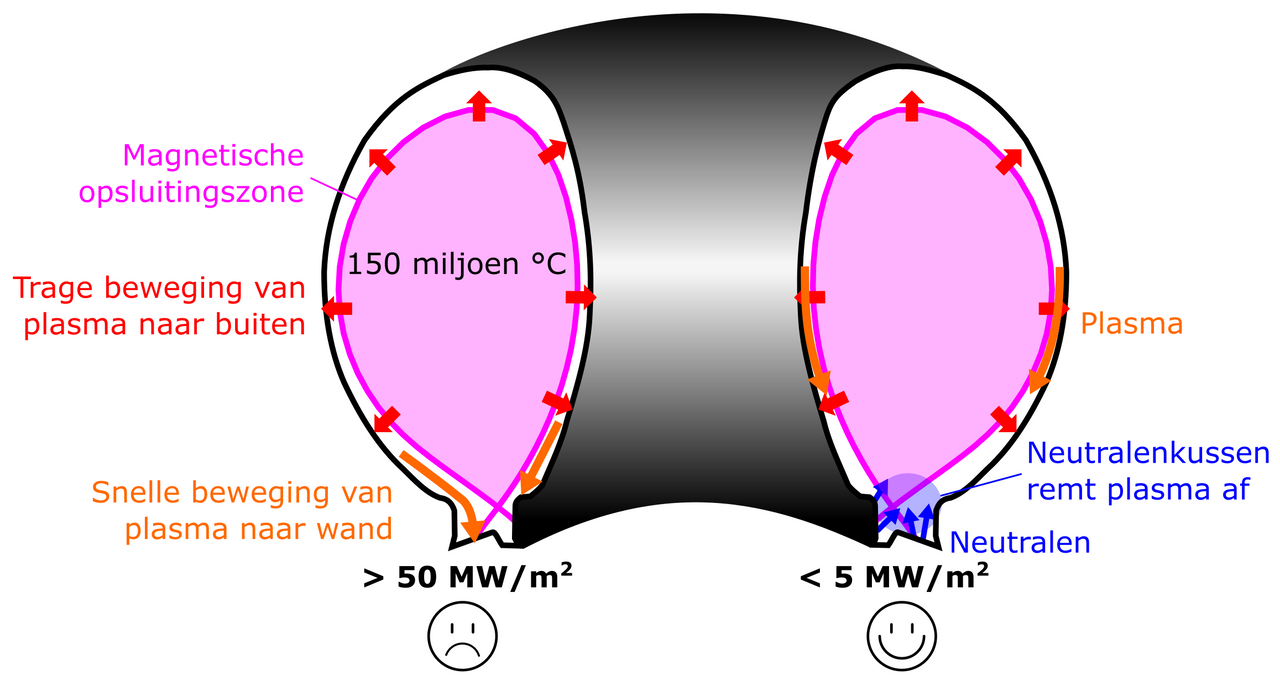
Fortunately, physics can help. When ions and electrons reach the reactor wall, they combine and reflect off as uncharged atoms and molecules (Neutral). This creates a neutral wall pad that can reduce the thermal load to less than 5 MW/m.2 (Right section position). You can compare this to holding a sponge under a running faucet. The water now comes out of the sponge from all sides and is distributed over a much larger surface area. Because Neutral It emits visible light, and this neutral plate is also visible on the right side of the opening image. With a thermal load of less than 5 MW/m2 and sufficient cooling around the reactor, the wall does not melt.
The role of computer models
The challenge is that the properties of this neutral pad depend heavily on the reactor design. For example, the shape of the reactor wall and the magnetic field affect the thermal load. Simulations using computer models support the design of future reactors. Known physics is poured into mathematical models that can be solved by computers. This way you get a digital replica of a nuclear fusion reactor, which we can experiment with without catastrophic consequences.
You can get the most accurate results by tracking all the individual particles (ions, electrons, atoms, and molecules) in the reactor using a computer. This is usually about 1023 Particles. The computation time per particle is easily over a minute, giving a total computation time of 1023 A minute = 200 quadrillion years (2 with 17 zeros). Even if you put thousands of computers together to do the calculations, you wouldn’t get any results before the sun burned out long ago.
Fortunately, a selection of about a million particles already provides a fairly accurate picture. If 1,000 computers did the calculations together, you would get the results within a day. These particle models are known as Kinetic models (Left side of figure below.) Assuming one minute of computation time per particle is an underestimate, and it often takes days or even weeks to get sufficiently accurate results. Kinetic models It is therefore intended to obtain accurate reference solutions, but is not suitable for design calculations that require regular repetition of simulations with different parameters.
Continuity models predict convection in less than a day of calculation time.
In our research group we develop models to determine the collective behavior of molecules (with a particular focus on Neutral) for description, known as Continuity models (Right side of figure below.) By cleverly grouping the particles, we can create equations for their average properties. Density tells how many particles are in a given location, flow rate describes their average speed of movement, and temperature gives an indication of their average kinetic energy. This Continuity models It usually provides a fairly accurate approximation of convection and is at least 1000 times faster than Kinetic modelsAllowing us to get results within one day using just one computer.
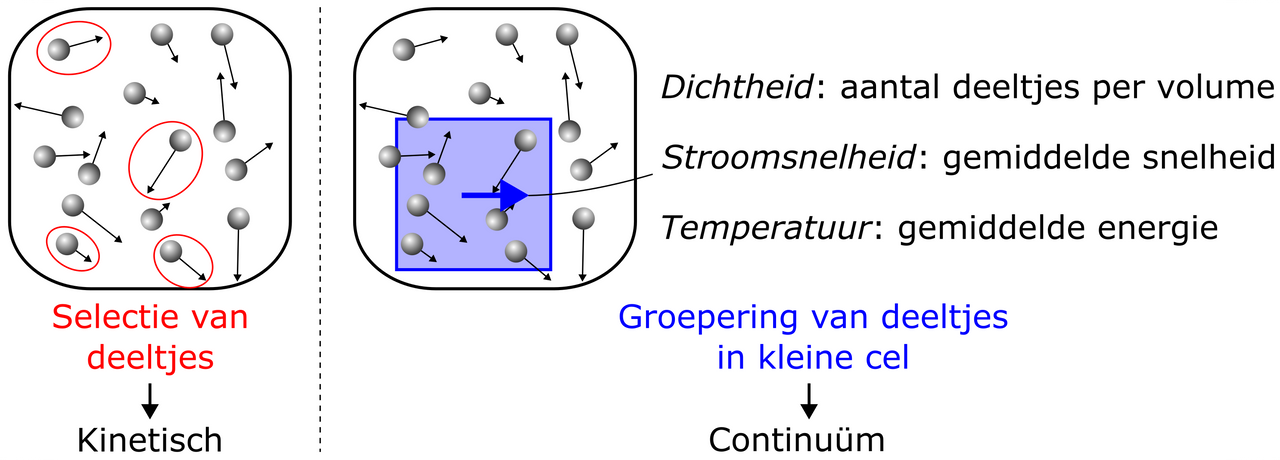
Will nuclear fusion for electricity production remain a thing of the future forever?
Nuclear fusion faces many technological challenges. In current experiments, the energy released during nuclear fusion is still less than the energy needed to heat the plasma. ITER (ITER – The Road to New Energy), the reactor currently under construction in France and scheduled to start operating in 2034, is designed to produce 500 megawatts of fusion power with an input power of 50 megawatts. In addition, more and more nuclear fusion startups are emerging. Electricity-producing reactors may not be available until the second half of 21is Whether the technology will finally make a breakthrough depends on how much design cost improves and on the value society places on the environmental footprint of electricity production.
Computer modeling has contributed to tremendous progress in nuclear fusion research and will continue to play a crucial role. Speeding up these models is essential to making them useful for design calculations. Continuity models It makes it possible to address the problem of huge heat loads in future reactors. By designing future reactors now, we can ensure that nuclear fusion will not always be around for thirty years.
More Stories
Which can cause an increase in nitrogen.
The Central State Real Estate Agency has no additional space to accommodate Ukrainians.
The oystercatcher, the “unlucky national bird,” is increasingly breeding on rooftops.